Soil Sampling as a Basis for Fertilizer Application
(SF990, Revised Sept. 2023)This publication outlines and explains reasons for soil sampling and methods to produce best results.
Importance of Soil Sampling
Soil testing measures the relative nutrient status of soils and serves as a foundation for profitable and environmentally responsible crop mineral nutrient application. Laboratory analysis quality control influences soil test value accuracy, but the quality of the soil sample may have an even greater influence on accuracy.
Sample collection is extremely important in the accuracy and repeatability of a soil test. Sample handling following field collection also is important. An unrepresentative soil sample may be misleading and may result in over- or underapplication of fertilizer. Therefore, collecting and handling soil samples properly is very important.

Sample Collection Timing
Soil samples for soil pH, zinc (Zn) and phosphorus (P) tend to be stable during the sampling year, which in North Dakota is usually from March through November. Potassium (K) values are cyclic through the growing season (Figure 1). The highest K values were in the early spring, and the lowest K values were in mid to late summer during dry soil conditions.
Many growers and landowners wish to track the trend of soil tests for several years. Therefore, obtaining soil samples for K analysis at the same time each year is important. Soil EC (electrical conductivity) is a measure for soluble salt content, and its value usually varies during the sampling season in response to rainfall and evapotranspiration.
Soil samples for nitrate-N analysis may be taken immediately after any grain harvest. Twenty years ago, NDSU recommendations included a sampling date adjustment for sampling fields before Sept. 15.
However, close inspection of data generated in North Dakota by sampling the same site from spring through fall, and then again the following spring, found that sites sampled after small-grain harvest sometimes increased in nitrate-N, sometimes decreased in nitrate-N and sometimes stayed the same. Therefore, the sampling date adjustment is not predictive and no sampling date adjustment has been included in NDSU fertilizer N recommendations since about 2005. Sampling behind the combine is more important.
Early sampling results in the ability to sample more fields due to more favorable sampling conditions (a better soil core) because fields are not tilled prior to sampling. Early sampling allows the soil test results to be used that year in fall N applications. Sampling before tillage also results in a much better 0- to 6-inch core for P, K and other nutrients and soil factors, rather than allowing tillage depth guesses to confound the depth.
Depth of Sampling
Soil sampling and analysis assumes 2,000,000 pounds per acre of soil from the 0- to 6-inch depth. This weight per unit volume (bulk density) value assumes a medium soil texture, with some minor compaction typically found following cropping and harvest activities.
Bulk density differences can make a difference of plus or minus 10 percent in soil test values. Bulk density is ignored in commercial soil sampling, but consistency in soil sampling techniques is important because of soil bulk density differences, particularly in soil cores. Sampling depth depends most on the nutrient or soil factor of interest, the crop to be fertilized and, in some cases, the tillage system in place at the time of sampling (Figure 2).

Nutrients
For soil pH, P, K, Zn, copper (Cu) and manganese (Mn), sampling the 0- to 6-inch depth is required because that is the depth of sampling related to crop response in this region. The 0- to 6-inch core is best obtained from land that has not been tilled yet.
If the field has been tilled, using a hand probe outside the vehicle, taking the sample in a spot where the sampler has firmed the soil with his/her feet, or taking the sample in the wheel track of the vehicle will result in a more consistent soil core. Otherwise, the loose soil may fall from the soil core or move out of the way of the soil core, and a 0- to 6-inch core will not be achieved. Instead, the core might represent a 2- to 8-inch depth, or a more random-depth soil, depending on the consistency of the loose soil.
For soil nitrate-N and chloride, a 0- to 2-foot core is required. Instead of analyzing just one 0- to 2-foot core, dividing the core into a 0- to 6-inch core and a 6- to 24-inch core, and analyzing each one separately would be instructive. Dividing the 2-foot core into 0- to 6-inch and 6- to 24-inch cores also will help with management of soluble salts (EC, electrical conductivity).
For sugar beet, malting barley and sunflower, a 2- to 4-foot soil core for nitrate-N also would help determine whether deeper soil nitrate might affect the N rate or crop quality (sugar concentration, grain protein and seed oil, respectively).
In sugar beet, many fields have been managed properly for many years, so the deeper soil nitrate-N is not greater than the 30 pounds of N per acre assumed to be present in most years at the 2- to 4-foot depth. Therefore, recently, these fields have not been sampled deeper than 2 feet. In fields new to sugar beet, we strongly encourage a 2- to 4-foot core.
For malting barley and sunflower, improved grain/seed quality and N recommendation management would benefit from a 2- to 4-foot soil core nitrate analysis; however, the areas in North Dakota where these crops are grown have many rocks or are in areas with numerous hidden pipelines, which often are not documented. Therefore, very few growers actually sample to the 2- to 4-foot depth, despite it being agronomically superior to the 0- to 2-foot depth alone.
The soil test procedure around the world and specifically in North Dakota for estimating soil sulfur (S) availability to crops for use in determining an S fertilizer rate is very poor, so no soil sampling should be conducted for S. Growers should refer to fertility publications for specific crops for information on how to approach S rates.
Crop
For crops that may require supplemental N, the nitrate-N analysis is conducted on a 0- to 2-foot core, or a 0-to 6-inch and 6- to 24-inch core. In sugar beet, the N recommendation also considers the 0- to 6-inch depth. If the N content of the 0- to 6-inch depth is less than 65 pounds of N per acre, fertilizer is applied to achieve 65 pounds of N per acre, regardless of what is in the 6- to 24-inch or greater soil depths.
For soybean, sampling for soil nitrate is not common; however, in areas of eastern North Dakota where iron deficiency chlorosis (IDC) is common, sampling for soil nitrate may help management of fields due to the effect that residual soil nitrate has on IDC severity.
Tillage System
Under conventional tillage and under conservation tillage that leaves residue but results in tillage deeper than 3 inches, the 0- to 6-, 6- to 24-, 0- to 2- and 2- to 4-foot soil depths all may be appropriate. However, in no-till/strip-till systems, surface or near-surface application of fertilizer results in stratification of especially soil pH, P and K through time. Soil pH may decrease and become very acidic near the soil surface due to surface or near-surface application of urea and other forms of N fertilizer.
When soil bacteria transforms these ammonia-based fertilizers to nitrate, acid is released as a byproduct. The 0- to 2-inch depth of soil may become so acidic that crop growth and yield are reduced due to aluminum toxicity.
Therefore, in fields under no-till systems, the 0- to 2-inch depth should be separated and analyzed separately for soil pH, P and K in addition to analyzing soil at the 2- to 6-inch depth.
For other plant nutrients and soil factors in the field, a 0- to 6-inch depth sample also should be obtained and analyzed.
Ridge-till and Starter-band Considerations
Some farmers may use ridge-tillage systems. Deep cultivation during the growing season builds the ridges and they remain in the field following harvest and through winter. At planting, the top of the ridge is removed, exposing moist soil for seeding, and soil from the top of the ridge is moved into the row middles.
Starter fertilizer commonly is used at planting in North Dakota, and sometimes deep-placed fertilizer is applied directly under the ridge-top in the fall. Ridge-till should be sampled to 0 to 6 inches either side of the ridge and straight down into the ridge (Figure 3).

Fields with a history of band applications of high rates of P and K are special problems, especially where within-field P and K levels are important. When band rates greater than 30 pounds of P2O5 and/or K2O are used, a residual band effect usually remains for several years.
If the bands can be located, such as in the season following the first year of banding, the bands should be avoided. However, in most fields, avoidance is impossible.
A good way to sample for P and K in these fields is to sample in a transect across a row span. In North Dakota, high reproducibility of P levels has been achieved in zone sampling by obtaining eight to 10 soil cores in banded fields on a transect (Figure 4).

Sampling Tools
Soil is variable side to side and with depth. A proper soil sample is obtained from a uniform volume with the sampling tool from the top of the sample depth to the bottom. Wedge-shaped samples or a handful of soil from the soil surface, or even a handful from the surface and one at depth, will not provide consistent soil sample values.
The best soil sample is obtained using a soil probe (Figure 5). Hand and automated probes are available in the commercial market.

Select the probe that enables the user to sample at the desired soil depth. A hand-held soil probe is handy to take a 0- to 6-inch sample, but deeper sampling may require an automatic hydraulic soil probe. Hydraulic soil probes in this region usually are installed in pickup truck cabs or on the side of the pickup truck bed on the driver’s side to enable the most efficiency soil sample handling (Figure 6).

Lubricant | Organic Matter % | Nitrate-N | P | K | Fe | Mn | Zn | Cu |
---|---|---|---|---|---|---|---|---|
ppm | ||||||||
No lubricant | 1.67 | 1.4 | 14 | 249 | 11.4 | 1.5 | 0.8 | 1.7 |
WD-40 | 1.59 | 1.3 | 16 | 248 | 13.2 | 1.8 | 1.0 | 2.0 |
PAM | 1.66 | 2.1 | 16 | 263 | 13.5 | 3.8 | 1.1 | 2.3 |
Dove dishwashing liquid | 1.67 | 2.6 | 14 | 280 | 10.1 | 1.3 | 0.7 | 1.2 |
Motor oil | 1.63 | 1.6 | 16 | 265 | 12.5 | 1.4 | 0.9 | 2.0 |
Silicone | 1.62 | 1.3 | 16 | 246 | 9.9 | 1.3 | 0.6 | 1.0 |
LSD 5% | NS | NS | NS | NS | 0.7 | 0.8 | 0.2 | 0.3 |
Soil Sample Handling
Samples intended for nitrate-N analysis should be stored in ice chests/coolers during transport. Moist samples subjected to heat will result in N release through microbial mineralization, and the soil test N values will increase during transport and storage. Samples intended for nitrate-N analysis should be air-dried immediately after collection to prevent alteration of nitrate-N concentrations due to microbial activity. The sample cores should be broken apart gently and spread onto a clean drying tray or clean paper in a dust-free area.
Another procedure would be to transport the soil samples directly to the soil testing laboratory in an ice chest/cooler, where the drying and subsequent soil preparations will be conducted immediately after documentation. The laboratory may impose a drying fee on samples submitted in a moist condition.
The person collecting soil samples intended for chloride analysis should wear rubber gloves to avoid body-salt contamination. Samples intended for Zn analysis should not come into contact with any galvanized surface, including the soil sampling tool, a bucket, drying container or grinder.
Soil Sample Collecting: Where and How
Where to collect soil samples from a field and how many samples are required depend on the goal of sampling. In North Dakota, many fields were sampled in the past as whole fields using a composite sample approach.
In the composite approach, 20 to 30 soil cores were collected in “representative areas” of the field and excluded any odd soils. The composite cores were mixed thoroughly. Then a subsample was taken from the mix and sent to the laboratory for analysis, resulting in one suite of soil test values that represented most of the field.
This approach was appropriate for a time before the ability to manage a field site-specifically, and to fertilize in an era of stable crop prices and low fertilizer costs. All of those assumptions have changed during the past 20 years; growers now have the ability to site-specifically manage fertilizer within fields, and crop prices and fertilizer costs are highly volatile. The usefulness of managing a field based on one soil test value per nutrient has diminished greatly.
Sampling for Within-field Soil Test Values
Sampling for within-field soil test values can be accomplished through grid or zone sampling. Grid sampling can reveal soil test value patterns, provided the sampling is conducted in a dense grid of one sample per acre (Franzen and Peck, 1995).
The pattern of grid sampling should be a systematic, unaligned grid as illustrated in Figure 7. Extensive research in North Dakota has indicated that any grid density less than this, such as one sample per 2.5 acres, is inadequate to reveal actual soil test patterns in fields.
Sampling using a one-sample-per-acre grid is expensive, but crop consultants sometimes use it when a client field is sampled the first time to verify that other sampling methods are similarly revealing.
Grid sampling is best conducted using the systematic, unaligned grid approach. In central Corn Belt states, a 2.5-acre grid usually is recommended because field P and K variability is mostly in the high soil test range, so whether the 2.5 acre grid delineates patterns is of no practical consequence because the recommendation within the different patterns is the same P and K rate.
Soil test P and K values in North Dakota form patterns based on topography due to more conservative historic rates of P and K in the region, compared with those in the central Corn Belt, so a 2.5-acre grid is inadequate for North Dakota grower needs (Franzen et al., 1998). Grid sampling is recommended in North Dakota only in areas with large manure applications or in fields that have received large amounts of buildup P and K fertilizers.

Zone Sampling
Zone sampling is based on the principle that the soil fertility/soil factor patterns in a field are related to some logical, more easily/practically measured property. Zone sampling research in North Dakota began in 1995, when a field near Valley City that was sampled in a quarter-acre grid to 4 feet in depth showed similar patterns after sunflower harvest in 1995, compared with the patterns observed after spring wheat in 1994 (Figure 8).

Similar patterns in this field were observed each year for 10 years. The nitrate-N values within a zone varied every year, but the pattern remained the same. In all of the fields sampled from 1994 to 2003, totaling more than 50 site-years, patterns were stable in fields between years.
Zones should be developed using at least two tools (Franzen et al., 2011). Aerial imagery from piloted aircraft or drones (unmanned aerial vehicles or UAVs) from growing crop canopies (Figure 9); satellite imagery of growing crops (Figure 10); soil electrical conductivity sensors (Figures 11 and 12); soil electromagnetic sensors (Figure 13); multiyear yield maps (Figure 14); topography developed from subinch accuracy GPS elevation (Figure 15), such as that available with an auto-steer system; and bare soil imagery from Farm Service Agency photographs may be used to help develop zones for use in soil sampling.








Soil type maps from the Natural Resources Conservation Service (NRCS) through published soil surveys or the web soil survey should not be used to develop site-specific zone maps due to the coarse nature of soil series delineation. Soil surveys are categorized as Order 2 by the NRCS and only recognize features that are at least 2.5 acres in size. A soil survey would need to recognize features no larger than 1 acre in size to be useful in delineating sampling zones (Franzen et al. 2002).
More zone development information is available in NDSU Extension publications “Developing Zone Soil Sampling Maps” (SF1176-2) and “Yield Mapping and Use of Yield Map Data” (SF1176-3) (Franzen 2018a, Franzen 2018b).
Sampling Within a Zone
One sample within a zone is not appropriate to represent a soil test value for the zone. Multiple cores must be obtained (Table 2). Generally, eight to 10 cores should be obtained randomly within a zone for the value to be reproducible.
Number of sample cores used to estimate the sampling area mean | ||||||
---|---|---|---|---|---|---|
Site | Mean lb/acre | 1 | 3 | 5 | 8 | 10 |
percent of composite values within ±20% of the mean | ||||||
1 | 16 | 26 | 44 | 50 | 62 | 62 |
2 | 55 | 0 | 52 | 70 | 86 | 88 |
3 | 61 | 30 | 56 | 78 | 86 | 92 |
4 | 28 | 54 | 82 | 90 | 98 | 98 |
5 | 12 | 52 | 78 | 90 | 96 | 98 |
References
Blaylock, A.D., L.R. Bjornestad and J.G. Lauer. 1995. Soil probe lubrication and effects on soil chemical composition. Communications in Soil Science and Plant Analysis 26: 1687-1695.
Breker, J.S. 2017. Recalibration of soil potassium test for corn in North Dakota. M.S. thesis, North Dakota State University, Fargo, N.D.
Franzen, D. 2018a. Soil sampling and variable-rate fertilizer application. NDSU Extension publication SF1176(2)
Franzen, D. 2018b. Yield mapping. NDSU Extension publication SF1176(3)
Franzen, D.W., and T.R. Peck. 1995. Field soil sampling density for variable rate fertilization. Journal of Production Agriculture 8:568-574.
Franzen, D.W., and D. Berglund. 1998. Small-scale spatial variability of soil N and P and implications with regard to sample core number. pp. 143-153. In 1997 Sugarbeet Research and Extension Reports. Vol. 28. NDSU Extension Service, Fargo, N.D.
Franzen, D.W., D.H. Hopkins, M.D. Sweeney, M.K. Ulmer and A.D. Halvorson. 2002. Evaluation of soil survey scale for zone development of site-specific nitrogen management. Agronomy Journal 94:381-389.
Franzen, D.W., D. Long, A. Sims, J. Lamb, F. Casey, J. Staricka, M. Halvorson and V. Hofman. 2011. Evaluation of methods to determine residual soil nitrate zones across the northern Great Plains of the USA. Precision Agriculture Journal 12:594-606.
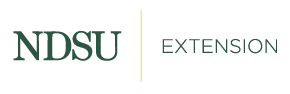
May 2018 (Revised May 2018)